Jiawu Zhao1, Lawrence Quach1, Clare Carney1
Swansea Medical School, Swansea University, Swansea, UK
Immune privilege and its role in the eye
150 years ago, van Dooremaal noticed that mouse skin transplanted onto the anterior chamber of a dog eye survived for a prolonged time (1). This observation occurred long before the mammalian immune system had been characterised. The term immune privilege (IP) however was coined by Sir Peter Medawar in the 1940s whose experiments showed acceptance of skin allografts placed in the anterior chamber of rabbit eyes (2). Now we understand that IP is a modified or reduced immune response by a specific tissue microenvironment.
In our bodies, there are several tissues that can limit their inflammatory reactions. These tissues are, the eye, brain, testes, placenta, and foetus (3, 4). However, the question is, why does IP exist in these tissues specifically? Taking the eye as an example, its function relies on the clarity of the visual axis and constituent tissues such as the corneal endothelium and some elements of the retina, which are amitotic and unable to regenerate. Thus, collateral damage from inflammatory responses would lead to severe consequences (5).
In contrast, tissues such as skin and mucosal surfaces, can generate strong immune responses to pathogens as well as experience extensive tissue damage and is also able to repair itself- processes that would not compromise their functionality (3). Therefore, it is believed that our body has developed IP for certain tissues through evolution.
Immune status of the eye
Although the eye is considered an immune privileged site, it is important to emphasise that this concept only applies to the inside of the eyeball, including the anterior chamber, vitreous cavity and sub-retinal space. On the outside of the eyeball, mucosal immunity plays a crucial role to fight off infection through immunoglobulins (mainly IgA) in the tear film and other immune cells such as macrophages, natural killer cells, dendritic cells, B cells and T cells. In addition, it has been found that lacrimal acinar cells are able to secrete lysozyme, lactoferrin, defensins and cytokines which also have anti-microbial functions (6).
Inside the eyeball, IP is maintained by two mechanisms. The first mechanism relies on the eye’s unique anatomical structure that separates it from the rest of the body. This mechanism is achieved passively through avoidance of exposure to danger. The second mechanism is the eye’s immunosuppressive microenvironment. This mechanism is achieved both actively through resistance of activation of immune responses and passively through tolerance of potentially dangerous signals (7, 8). Our current understanding of IP mechanisms is described below and outlined in the Figure 1.
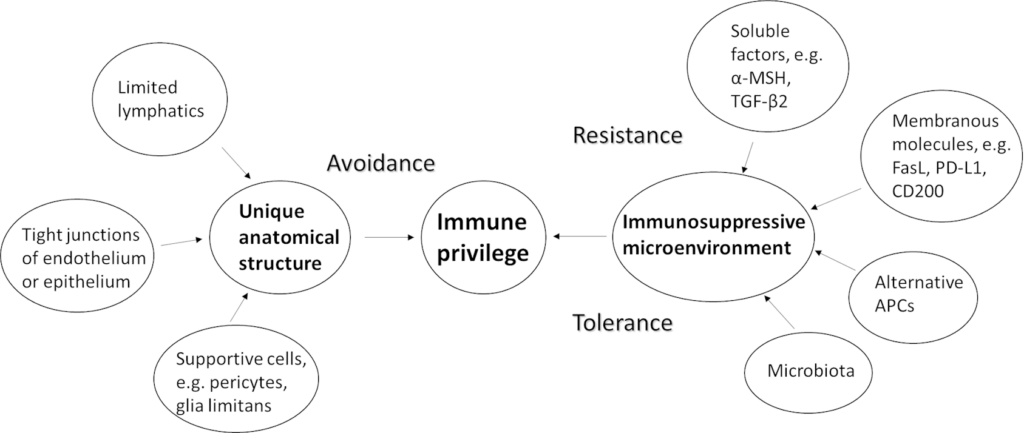
Mechanisms of immune privilege in the eye
The eye’s unique anatomical structure provides one of the two mechanisms of IP in the eye. So, what is unique about the eye’s anatomical structure? Firstly, there is limited lymphatic drainage system inside the eyeball. Recently identified markers only revealed lymphatics in ciliary body. They act as a pathway to drain aqueous humour (9). Secondly, the endothelium of blood vessels in the eye is non-fenestrated due to tight junctions. This means that extravasation of immune cells and macromolecules are limited (5). Further studies reveal that such a blood-retinal barrier (BRB) is not just a physical barrier of endothelial cells bound together by tight junctions, but also contain a wide range of immune cells such as pericytes, perivascular macrophages, glia limitans (foot processes of astrocyte/Müller cells) which can kill pathogens (3). In addition to endothelial cells, retinal pigment epithelial cells also form tight junctions to separate the retina from the highly vascularised choroid (10). The second mechanism of IP relies on the immunosuppressive microenvironment in the eye. Such a microenvironment is created by some soluble factors, membrane bound molecules, and alternative antigen presenting cells (APC) (11).
The immunosuppressive phenomenon of aqueous humour has been known for a long time. This phenomenon was shown both in vivo (12) and ex vivo (13) which prompted scientists to think what soluble factors in aqueous humour were causing these immunosuppressive effects. For the past couple of decades, alpha-melanocyte-stimulating hormone (α-MSH) and transforming growth factor-beta2 (TGF-β2) were the most extensively studied soluble factors in aqueous humour. It was found that neuropeptide α-MSH can mediate induction of CD4+ T regulator cells along with TGF-β2 mediated suppression of effector T cell activity (10, 14). In addition, it has been found that this immunosuppressive effect is enhanced by several other soluble factors in aqueous humour, such as vasoactive intestine peptide, somatostatin, and retinoic acid (10, 14). Therefore, we can consider the aqueous humour as a cocktail of different immunosuppressive factors. Further studies revealed that pigmented cells in the iris, ciliary body and the back of retina were also sources of these soluble factors (10). Pigmented cells in the iris also express the cell surface protein CD86 to dampen T-cell activation and retinal pigment cells express the protein programmed death-ligand 1 (PD-L1) to induce apoptosis in immune cells via (10). Moreover, endothelial cells of the cornea also express FasL and PD-L1 to induce apoptosis in activated T cells with FAS or PD expression (14).
In the retina, microglial cells are macrophage-like APCs. They express the membrane glycoprotein CD200L which is bound by CD200 on retinal neurons and behave like a myeloid-derived suppressor cell. Without this kind of anti-inflammatory signalling, the eye is more susceptible to uveitis, as demonstrated in animal models (15). Roles of the other cell surface molecules have been reviewed by Molzer et al (3). Absence of certain surface molecules also contributes to the eye’s immunosuppressive mechanisms. For example, corneal endothelial cells and neural retinal cells express very little MHC class I molecules responsible for presenting viral or endogenous antigens to CD8+ cytotoxic T cells. Therefore, the eye can avoid the destructive effects of cytotoxic T cells (16).
Alternative APCs refer to APCs primed in the ocular microenvironment. They first move to the thymus to promote thymocyte generation, and then to the spleen to induce immune tolerance- rather than an inflammatory response. Hence, they are also known as tolerogenic APCs. In general, alternative APCs suppress Th1 and Th2 differentiation and B cell activation, and induce Treg development, cells which are crucial in maintaining immune tolerance and preventing autoimmunity. It has been found that macrophages activated by TGF-β in the aqueous humour leads to increased IL-10 expressing T cells (17). Depending on the origin of the alternative APCs, this process can be anterior chamber associated immune deviation (ACAID), vitreous cavity associated immune deviation (VCAID), or sub-retinal space associated immune deviation (SRSAID) (8).
A recent study found that, in addition to suppress pathogenic settlement, the homeostatic microbiome contributes to induction of Treg from either naïve T cells or anergic T effector cells via its priming effect on residential dendritic cells. It has been shown that such priming effect depends on the nutrients and metabolites generated by the microbiota. Also, homeostatic microbiome plays an important role in establishing the BRB. The healthy BRB prevents infiltration of autoreactive T cells and retinal inflammation. If the microbiome is dysregulated (dysbiosis), T cells have the potential to cross the BRB and react with retinal antigens and initiate inflammation (3).
How can we utilise the concept of immune privilege in the eye to benefit us?
First, IP can help us to improve the survival rate of transplantation. Patients with previous ocular allergies experience increased corneal graft rejection. Pre-clinical studies have shown that IP mechanisms can be used to promote allograft survival. For example, eye drops containing α-MSH are used prior to corneal allograft transplantation (18), or low-dose IL-2 is used to expand Treg function systemically and reduce immune cell infiltration of the allograft (19).
Secondly, novel therapies based on our current understanding of IP can help us to treat ocular inflammation that are caused by primary autoimmune conditions such as Sjӧgren’s syndrome or by anti-cancer immunotherapy such as ipilimumab (anti-CTLA4 antibody) and nivolumab (anti-PD-1 antibody) (10, 20).
Moreover, it has been envisaged that IP mechanisms or cells of immune privileged tissues can be used to create a localised IP site in other tissues to promote transplantation or treat autoimmune conditions. In this way, patients can avoid long-term immunosuppressants or steroid treatments (14). Still, there is so much more to understand about the mechanisms and nature of IP not just in the eye, but in other tissues too.
References
1. van Dooremaal JC. Die Entwicklung der in fremden grund versetzten lebenden geweba. Albrecht von Graefes Arch Ophthalmol. 1873;19:358-73.
2. Medawar PB. Immunity to homologous grafted skin; the fate of skin homografts transplanted to the brain, to subcutaneous tissue, and to the anterior chamber of the eye. Br J Exp Pathol. 1948;29(1):58-69.
3. Molzer C, Heissigerova J, Wilson HM, Kuffova L, Forrester JV. Immune Privilege: The Microbiome and Uveitis. Front Immunol. 2020;11:608377.
4. Boyd K. The Eye and Immune Privilege 2018 [Available from: https://www.aao.org/eye-health/tips-prevention/eye-immune-privilege.
5. Niederkorn JY. The Eye Sees Eye to Eye With the Immune System: The 2019 Proctor Lecture. Invest Ophthalmol Vis Sci. 2019;60(13):4489-95.
6. Nieto-Aristizabal I, Mera JJ, Giraldo JD, Lopez-Arevalo H, Tobon GJ. From ocular immune privilege to primary autoimmune diseases of the eye. Autoimmun Rev. 2022;21(8):103122.
7. Taylor AW. Ocular immune privilege. Eye (Lond). 2009;23(10):1885-9.
8. Chen M, Luo C, Zhao J, Devarajan G, Xu H. Immune regulation in the aging retina. Prog Retin Eye Res. 2019;69:159-72.
9. Nakao S, Hafezi-Moghadam A, Ishibashi T. Lymphatics and lymphangiogenesis in the eye. J Ophthalmol. 2012;2012:783163.
10. Keino H, Horie S, Sugita S. Immune Privilege and Eye-Derived T-Regulatory Cells. J Immunol Res. 2018;2018:1679197.
11. Hori J, Yamaguchi T, Keino H, Hamrah P, Maruyama K. Immune privilege in corneal transplantation. Prog Retin Eye Res. 2019;72:100758.
12. Cousins SW, Trattler WB, Streilein JW. Immune privilege and suppression of immunogenic inflammation in the anterior chamber of the eye. Curr Eye Res. 1991;10(4):287-97.
13. Kaiser CJ, Ksander BR, Streilein JW. Inhibition of lymphocyte proliferation by aqueous humor. Reg Immunol. 1989;2(1):42-9.
14. Taylor AW. Ocular Immune Privilege and Transplantation. Front Immunol. 2016;7:37.
15. Copland DA, Calder CJ, Raveney BJ, Nicholson LB, Phillips J, Cherwinski H, et al. Monoclonal antibody-mediated CD200 receptor signaling suppresses macrophage activation and tissue damage in experimental autoimmune uveoretinitis. Am J Pathol. 2007;171(2):580-8.
16. Le Bouteiller P. HLA class I chromosomal region, genes, and products: facts and questions. Crit Rev Immunol. 1994;14(2):89-129.
17. Niederkorn JY. The induction of anterior chamber-associated immune deviation. Chem Immunol Allergy. 2007;92:27-35.
18. Hamrah P, Haskova Z, Taylor AW, Zhang Q, Ksander BR, Dana MR. Local treatment with alpha-melanocyte stimulating hormone reduces corneal allorejection. Transplantation. 2009;88(2):180-7.
19. Tahvildari M, Omoto M, Chen Y, Emami-Naeini P, Inomata T, Dohlman TH, et al. In Vivo Expansion of Regulatory T Cells by Low-Dose Interleukin-2 Treatment Increases Allograft Survival in Corneal Transplantation. Transplantation. 2016;100(3):525-32.
20. Hori J, Kunishige T, Nakano Y. Immune Checkpoints Contribute Corneal Immune Privilege: Implications for Dry Eye Associated with Checkpoint Inhibitors. Int J Mol Sci. 2020;21(11).